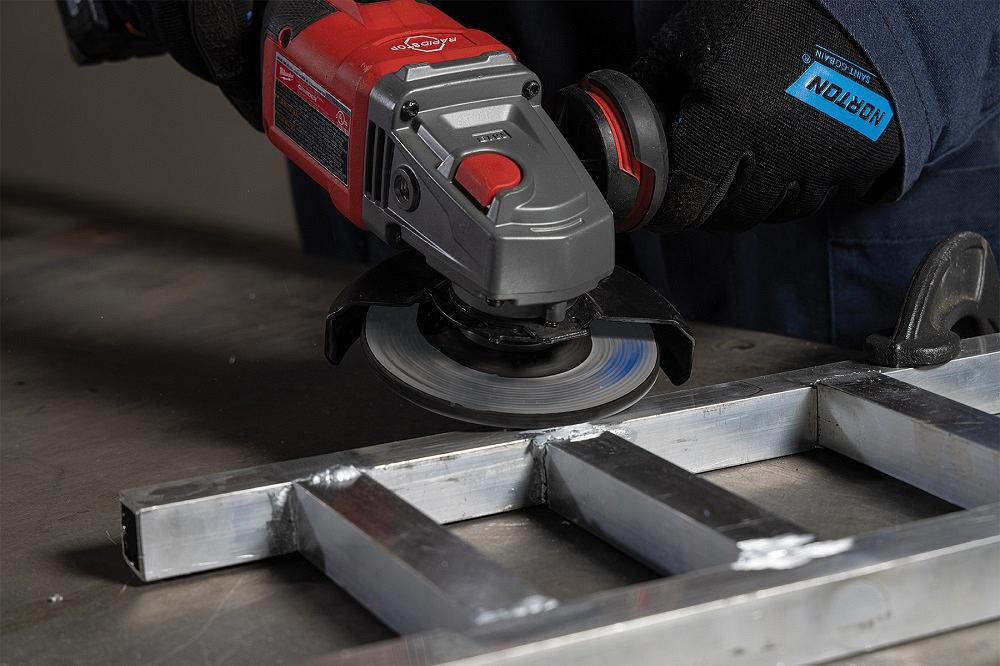
Manual grinding of aluminum parts can be challenging. Using an abrasive specifically designed for the task will give the best results.
In recent years, the transportation industries have increasingly turned to aluminum as they look to “lightweight” their products. As a result, metal fabricators need new tools to work with this challenging material.
Aluminum alloys offer an improved strength-to-weight ratio compared to traditional steel alloys. Lightening trends in the transport sector lead to the need for fast and efficient tools to straighten aluminium. Typical right-angle wheels designed for steel are not used on aluminum because the surface of the wheel can quickly become clogged with metal chips that stick to the abrasive.
By investigating the mechanisms by which metal shavings can become attached (loaded) to the abrasive wheel surface, strategies to avoid metal loading can be applied to the abrasive design. This then leads to new aluminum grinding products with greatly improved grinding speeds and longer lasting performance, without the need for waxes or lubricants.
Increase the use of aluminum
Aluminum used in industry is usually not the pure element, but one of a number of aluminum alloy families, depending on the end use. Although the properties of specific aluminum alloys can vary widely, it is safe to make the following generalizations:
- Aluminum alloys are much lighter, only about one-third the density of steel alloys (2.7 g/cc vs. 7.85 g/cc).
- Although they are not as mechanically strong as steel, their strength-to-weight ratio is higher.
The production and use of aluminum is increasing. Although total steel production is higher than aluminum production today, the growth rate of aluminum production is about 60% higher than that of steel. From 2008 to 2018, global aluminum production grew at a compound annual growth rate (CAGR) of 4.8%, while global steel production grew at a CAGR of 3.0%.
Growth in aluminum use is primarily driven by the automotive and transportation industries, followed by aerospace and defense and marine. The automotive and transportation industry accounts for about 80% by value of the global use of high-strength aluminum alloys with an estimated CAGR of 7.7% from 2018 to 2023.
The strong need for aluminum in the automotive and transportation industry is driven by trends in lightweighting. Automakers are under constant pressure to increase the fuel efficiency of their fleets, so they naturally look to stronger and lighter materials. In the commercial trucking industry, lighter trailers can result in more cargo weight transported per trip, as well as fuel savings. Aluminum is also used to reduce the weight of marine vessels, which helps their speed, maneuverability, stability and fuel economy. Lightweight hulls also allow for operation in shallow water.
The challenges of working with aluminum
Aluminum alloys also have lower hardness, higher ductility, and lower melting points compared to steel alloys (932 degrees F to 1,112 degrees F for aluminum versus about 2,732 degrees F for steel). These differences can mean that the metalworking tools and techniques used for steel work are not always optimized for aluminum work.
A common problem when hand grinding aluminum parts is the tendency for aluminum shavings to stick to the same grinding wheel. When the wheel becomes loaded (clogged) with metal shavings, it cannot remove more metal from the workpiece. figure 1 shows a standard grinding wheel after a few minutes of use in aluminum. Since this wheel was designed to be used on steel, not aluminum, a load occurred and the wheel effectively stopped grinding.
A practice that delays the onset of metal loading is the application of wax to the grinding wheel. By applying a slippery substance to the surface of the wheel, it temporarily hinders the adhesion of the aluminum chips. However, as the wheel is used, the wax wears off and must be reapplied. This option is not ideal, as the application of wax takes the time of grinding and creates additional contamination on the workpiece, which must be cleaned when the grinding is finished. If the wax is not thoroughly cleaned from the workpiece, it can cause defects in the weld.
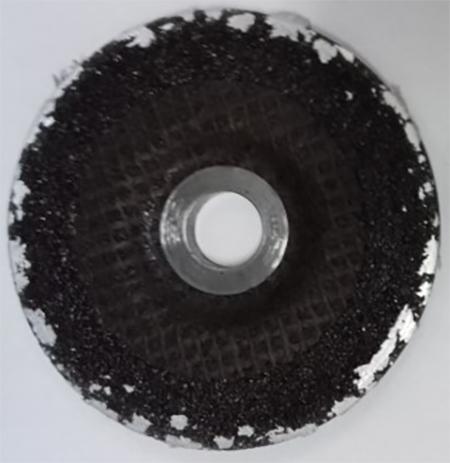
FIGURE 1. This is an example of a standard grinding wheel, not specifically designed for use on aluminum, after it has been used to grind aluminum. Notice all the silver colored (charged) areas of stuck metal, rendering the grindstone ineffective.
A high-magnification camera focused on charged areas on the surface of a used grinding wheel (see Figure 2) reveals an abrasive surface that cannot do the job it was designed to do. The bright areas are the aluminum metal glued to the face of the wheel. The white, blocky features are the abrasive grain. The yellow area is the exposed and worn areas of the bond, and the brown is the underlying bond and pores.
The image on the right of Figure 2 shows the cutting point of a single grain, the surface of which has been coated with aluminum metal. Behind the cutting point there are many stringy aluminum chips that have been collected. Since these chips were not being removed from the grinding zone, they were fused together by the friction and heat generated when the grain hits the workpiece. The streaks in the center of this mass show rubbing marks between the piece of aluminum and the aluminum attached to the grinding wheel. As the aluminum collected on the face of the grinding wheel, it blocked the cutting tip from removing more chips, obstructing the metal removal process.
A cross-section of this wheel (see Figure 3), viewed with a microscope, reveals the metal charge from a side view.
Close examination by a scanning electron microscope of aluminum chips removed from the surface of this wheel reveals even more (see Figure 3, right). The close focus of the top of the chips shows rub/plow marks, suggesting semi-solid behavior. The bottom of the chip shows how the aluminum was able to deform and stick to the entire surface of the wheel, adapting to both the grain and the bond. These deformation characteristics show that the metal softened near its melting point as it bonded to the wheel surface and that the mass grew cohesively as other aluminum shavings stuck together.
Figure 4 shows a framework of how the abrasive grain, the bond holding the grain, and the workpiece being ground can interact in the processes of cutting (removing material), plowing (moving material), and sliding (modifying the surface). The features observed on the wheel surface are mainly indicative of sliding interactions from when the abrasive grains came into contact with the aluminum part. The sliding interactions do not contribute to the metal removal process (chip formation) and only act to make the grinding process less efficient.
During aluminum grinding (see Figure 4), the grain is plowing through the ductile part, which covers the tips of the metal grain. Once the grain tip is coated, the frictional interactions between the chip (attached to the grain) and the workpiece allow the bonded metal chip to begin to grow cohesively. As the bonded metal patch grows, more interactions between the bond and the workpiece generate more heat, resulting in a larger area affected by the metal loading.
During use, as the abrasive wheel becomes clogged with metal, the grind becomes less efficient, causing the operator’s natural reaction to push harder with the grind to try to break up the wheel even more and open the surface to expose new cutting grains. However, this common approach does not work, as increased grinding pressure leads to a build-up of more heat, which continues the process of softening and sticking the aluminum shavings to the face of the wheel. This creates a feedback loop, which acts as a vicious cycle to further stress the wheel until it can no longer be ground and needs to be replaced.
New abrasive technology for aluminium
To break the feedback loop of the loading mechanism, the abrasive grain must be more resistant to metal loading. This is because the loading mechanism starts at the grain tips and grows cohesively to cover large areas of the grinding wheel.
During grinding, the individual abrasive grains undergo thermal and mechanical stress as they continuously impinge on the workpiece. These stresses can cause the grain to crack or fracture in different ways (see Figure 5). The type of grain fracture as well as the overall rate of grain fracture depends on grain microstructure and correlates with various grain properties, including hardness and resistance to heat, impact, and shock. A grain that fractures and breaks easily is known as friable, and one that wears slowly is known as durable.
The grain fracture is self-sharpening as it exposes new cutting surfaces. In the case of aluminum grinding, as the grain is fractured, the ejected parts can lift off stuck pieces of aluminum metal, leaving behind a fresh, clean cutting edge.
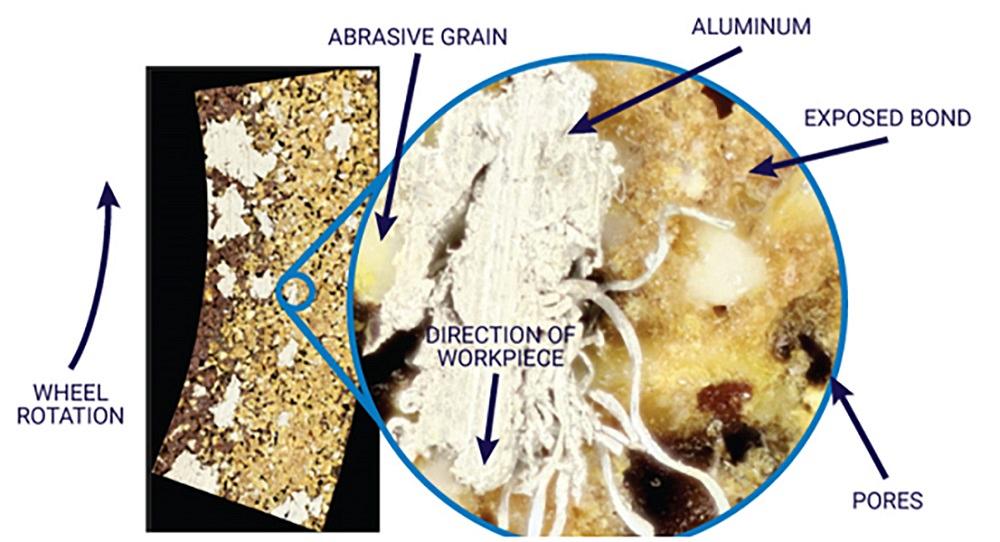
FIGURE 2. A top-down view of a used grinding wheel section is shown.
To demonstrate the effect of friability on grinding speed (metal removal rate) and loading extent, wheels containing grit types with different levels of friability were prepared and tested for grinding. All other experimental parameters were kept the same.
After the grinding tests were completed, each wheel bead was imaged to determine the extent of metal loading by calculating the total bevel area covered by the bonded metal (see Figure 6).
As a result, a strong correlation was found between grindstones containing highly friable grain types with less metal loading and higher grinding speed.
This has led to the development of aluminum grinding wheels with a special, extra-friable abrasive grain that is able to crack and break just before too much pressure and heat is generated, preventing the metal from building up (see Figure 7). These abrasive wheels are aggressive, which allows the manual grinder to work with less effort compared to using abrasive discs not specifically designed for aluminum removal.